Trial test
- Synthesis of nanoparticles such as oxides and composite oxides using supercritical water, subcritical water, and solvothermal method.
- Organic modification to the particle surface
- Decomposition using high temperature and high pressure water
- Active ingredient extraction, drying, washing, and impregnation using supercritical carbon dioxide
- Anhydrous cleaning of precision parts using dry snow, which is a fine dry ice cleaning system.
It is possible to visit for the experiment.
We have XRD and light microscope equipment.
It is proportional to the concentration and flow rate of the raw material, but in a typical example, it is 2 to 3 g / hour (MOMI-cho mini).
Please contact us. We will have a meeting.
There are numerous parameters that can be varied, including temperature, pressure, type of reaction solution, absolute and relative flow rates, flow type, and so on. It is impossible to cover all of these parameters in a single day of testing. We recommend the following approach:
*Regarding pressure, it is common to fix it at the maximum pressure of the equipment that is planned to be introduced in the future (except for batch experiments under the vapor pressure condition of subcritical water).
*The flow ratio is determined so that the temperature after mixing high-temperature water (supercritical or subcritical) with ambient-temperature feed reaches the target value. In cases where the temperature reaches 380°C after mixing, he flow rate of the raw material is often set to 10-30% of the supercritical water flow rate.
*The flow rate of high-temperature water is determined by the heater capacity rather than the pump capacity. If you want to use more than 10 ml/min of supercritical water, additional heaters are employed.
*Regarding the type of solution, it is advisable to consider the ion species and pH. For SUS316 piping, the pH range typically falls between 3 and 11. Additionally, chloride ions can strongly corrode SUS materials, so for acids, nitric acid or acetic acid is commonly used. Inconel-lined titanium piping is used under severe conditions, but it is not recommended due to the cost and lead time.
*In addition to aqueous solutions, slurries can also be used as feedstocks. For example, in the case of synthesizing oxide particles, hydroxide slurries are more effective than aqueous solutions in preventing pipe clogging. Since pipe blockage is a major challenge in particle synthesis, slurries are considered an important option.
*When using biomass slurries as feedstock, it is important to use smaller particle sizes and lower concentrations to minimize the risk of blockage, as larger particle sizes are highly susceptible to clogging.
*The temperature has the most significant impact on the reaction. Even a 50°C change can often lead to completely different results, making it a high-priority factor to consider. When investigating entirely unknown reactions, it is advisable to increase the temperature in 50°C increments as time allows.
*Regarding flow types, there are rapid heating, slow heating, and batch tests. Rapid heating involves mixing high-temperature water with room-temperature raw material liquid for instantaneous heating. Slow heating involves heating only the feed in the furnace without mixing, requiring a reaction tube coil. If a sufficiently long reaction time is desired, batch testing rather than flow testing is conducted.
*Although pure water is flowed for a certain period of time between different experimental conditions, this does not completely wash away the particles produced under the previous conditions. It is advisable to anticipate that the previous conditions will inevitably contaminate the subsequent conditions and design the experiment accordingly.
The solubility of a substance depends on temperature and density of carbon dioxide. At low density, it exhibits gaseous properties, and solubility decreases.
Therefore, higher pressure is generally better. However, large-scale equipment for pressures above 16 MPa requires different piping and other components, which can significantly increase the cost. Therefore, testing at the upper limit of 16 MPa is also common (the pressure resistance of the testing machine itself exceeds this limit).
Temperature is more complex. In general, the solubility of a substance increases with increasing temperature at a constant “density”. However, at a constant “pressure”, increasing temperature can lead to a decrease in density and, consequently, a decrease in solubility. A typical example is illustrated in the diagram. In many cases, the slope of increased solubility due to pressure rise becomes more significant at higher temperatures. This means that there is a pressure (10-30 MPa) at which the solubility at high temperatures exceeds the solubility at low temperatures.
Materials near miscibility with carbon dioxide may exhibit a sharp increase in solubility above a certain density. Therefore, it is sometimes sufficient to test at pressures slightly higher than the critical pressure (in which case, liquid carbon dioxide may also be considered as an option).
However, besides solubility, the effect of diffusion must also be considered. In the case of biological materials, it is often necessary to grind the material, which accelerates the diffusion of substances. While solubility represents the equilibrium state, diffusion relates to the rate of dissolution, which affects extraction time. Therefore, increasing the temperature is advantageous for accelerating diffusion.
In our test cases, there are instances where higher temperatures are beneficial, as well as cases where lower temperatures are preferable. This complexity arises from the interplay between the chemical properties of the substance and the structural characteristics of the material.
For the above reasons, we recommend fixing the pressure at the maximum of the planned equipment and varying the temperature, sample type, and presence or absence of an entrainer, unless the decision to use an introduction device above 16 MPa is a factor in the judgment.
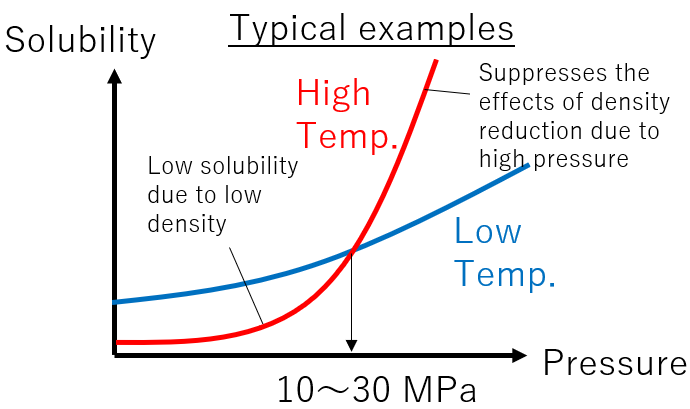